)
Modeled in 3D and validated using Computational Fluid Dynamics (CFD), the APR Performance GT-1000 Adjustable Wing is designed to adapt to a variety of widebody sports and touring car applications.
CFD Data & Analysis for the GT-1000 adjustable Wing
OVERVIEW
Contained herein are the data and results of the Computational Fluid Dynamics (CFD) analysis that was conducted on the a 3D model of the GTC-300 airfoil. This data illustrates how the airfoil performs in free-stream air flow by comparing Downforce vs. Angle-of-Attack (AOA) vs. Speed, and Drag vs. AOA vs. Speed. This data will provide insight with regards to how the airfoil performs with respect to these conditions. Additionally, the analysis contained herein will provide insight with regards to how to apply this data to real world applications.
DEFINITIONS
Typically, the AOA is defined as the difference in angle between the chord line and the vector that represents the undisturbed free-stream air flow, where the chord line is the line that passes from the leading edge to the trailing edge of the center section of the airfoil. For the CFD simulations conducted on the GTC-series airfoils, the center cross-section of the reference plane (or "center reference line") is used in place of the "chord line." Therefore, the AOA is defined herein as the difference in angle between the center cross-section of the reference plane (shown in the image below) and the vector that represents the relative motion of the undisturbed free-stream air flow. A ruler that is placed on top of the center section of the GTC-series airfoil would be the real-life equivalent of this reference line.

DATA COLLECTION METHODOLOGY
CFD simulations were conducted on a range of AOA values from 0 to 15 degrees, and speeds of 80 to 165 mph (approx 128 bis 266 km/h). Data values gathered from 18 different configurations were recorded using a combination 6 AOA values (0, 5, 10, 12, 13, and 15 degrees) and 3 free-stream air speeds (80, 100, and 120 mph (approx 128, 161 und 193 km/h)). Downforce (or "negative lift") and drag values are recorded for each configuration. The AOA values were increased by in increments of 5 degrees, with additional data taken at AOA values of 12 and 13 degrees (the reason for this will be discussed in a bit). Speeds of of 80 to 120 mph (ca. 128 bis 193 km/h) were used to represent medium-high vehicle speeds. Additionally, a speed of approximately 165 mph (ca. 266 km/h) was used to provide data for pressure distributions, vector fields, and streamlines.
INTERPRETING THE DATA
Caveat: The first thing we must take into account as a caveat, before attempting to make any sense of this data, is to realize that this all this data corresponds to a 3D airfoil placed in an environment of free-stream air. So what's the significance? The significance lies in the design and intended application of this particular airfoil. The chord length and chord line angle vary along its length in such a way as to be optimized for use at the rear of a typical sedan-shaped vehicle, where the roof top is taller than rear trunk area. Basically, this airfoil was never intended to be used in this CFD simulation's environment of free-stream air. This doesn't mean this data is useless. This just means that we must understand that we have certain limitations, and that application of this data must be applied to the real-world carefully.
AOA & Speed vs. Downforce & Drag: A quick scan of the data values shows that increasing the speed results in increase of both downforce and drag, and increasing the AOA (up to a certain point) also increases both the downforce and drag.
Variable Chord Line Angle: Starting at AOA = 0 degrees, the airfoil is already producing 672 N (or 151 lbf) of downforce at 80 mph (ca. 128 km/h), with a lift-to-drag (L/D) ratio of approximately 8.8. By looking at the pressure distribution rendering below, we can see that, in the free-stream air flow, the outer sections of the airfoil contribute more to the net downforce than the center section does. For reference, the outer sections of the GTC-300 airfoil can have a positive chord line angle up to 15 degrees with respect to the center chord line. So, most of that 151 lbf of downforce comes from the outer sections. Of course, this pressure distribution changes when the airfoil is applied to a real-world scenario (installed on a vehicle), where the center section would see a very different air flow than the one seen here.
Stall: At AOA = 10 degrees, we can see the in the graphs that the downforce begins to plateau. Between AOA = 10 and 12 degrees, there is very little gain in downforce, while drag continues to rise. L/D ratio varies from approximately 7.1 to 8.1. What is happening here is that the outer sections of the airfoil, which are now at an angle of 25 to 27 degrees (by adding 15 degrees due to the variable chord line angle) relative to the free-stream air flow, are beginning to experience air flow separation such that the downforce on the outer sections now decreases with increasing angle. The center section, being at an angle of 10 to 12 degrees relative to the free-stream air flow, continues to provide more downforce with increasing angle. The plateau in the downforce in this range indicates that the increasing downforce of the center section compensates for the decreasing downforce in the outer sections, such that the net downforce still increases with increasing AOA. What we are seeing here is the range of diminishing gains with respect to increasing the AOA.
More Stall: Between AOA = 12 and 13 degrees, we can see that the net downforce now decreases with increasing AOA, and that the L/D ratio has dropped below 6.4 in some cases. This indicates that the gradually increasing downforce in the center section is no longer able to compensate for the not-so-gradually decreasing downforce in the outer sections. Additionally, because of the further air flow separation experienced by the outer sections, the magnitude of drag begins to increase at a higher rate. The scenario here is now beyond what we would call diminishing gains. We are seeing significant losses. Aside from figuring out that it wouldn't be a good idea to operate the airfoil beyond this range of angles, we can note these important points:
1) The center section, at AOA = 12 to 13 degrees, is nowhere near its stalling range.
2) The outer sections, which are now at 27 to 28 degrees, are the sections that are operating in their stalling range.
3) Again, recall the caveat mentioned earlier --> That this data is derived from a free-stream air flow environment, that this airfoil was not designed to function optimally in a free-stream air flow environment, and that care must be used when applying these results in real-world application.
What's Not Shown in the Tables or Graphs: By now, we have already figured out that there really is no point to conduct CFD simulations on center section AOA values beyond 15 degrees. Without using up valuable CFD time, and knowing that the center section has similar of the camber to the outer sections (which are at +15 degrees greater angle than the center section), we can deduce that the decreasing downforce or increasing drag curves in the graphs would most likely increase in steepness significantly beginning in the AOA = 25 to 27 degrees range.
CFD DATA (TABLES)
The following tables show the actual data that were collected from the CFD analysis. The numbers in the table are represented in Newtons (a unit of force). To convert to "pounds," divide the numbers by 4.44822 (where 1 Newton (N) = 4.44822 Pounds-force (lbf)). For example, a downforce of 1286.89 N would equal 289.3 lbf.

CFD-Data (rendered images)

Connecting the Data to Real-World application
What we want to do now is to try to apply a bit of the CFD data and analysis to the real-world application of using this airfoil on a vehicle. First, we will first need to change a few things around. The first thing to change is the environment, because the mounted airfoil (both the center and outer sections) will never truly see any free-stream air flow. Even though the outer sections of the airfoil may be positioned beyond the vehicle's roof and body, and even though the air flow may be "cleaner" or be more parallel to the ground plane, the air around the sides of the vehicle is still affected such that it can no longer be considered to be free-stream air. Secondly, since we can no longer define angle with respect to the relative motion of free-stream air flow, it is helpful to introduce and use an additional term called "pitch." Previously, we had defined the AOA as the difference in angle between the center cross-section of the reference plane (a.k.a. "center reference line") and the vector that represents the relative motion of the undisturbed free-stream air flow. We will define pitch as the difference in angle between the center cross-section of the reference plane (a.k.a. "reference line") and a non-sloped ground plane that is parallel to the vehicle's direction of travel. A ruler that is placed on top of the center section of the GTC-series airfoil would be the real-life equivalent of the reference line. Additionally, to simplify the angle references and avoid confusion with AOA numbers, we will use only absolute values (i.e. positive numbers), in conjunction with either upward pitch (front higher than the rear) or downward pitch (front lower than the rear).
When the airfoil is mounted on the sedan-bodied vehicle, the center section actually be at an AOA that is greater than 0 degrees. This is because the air flow to the center section will have a tendency to follow the rear slope of the roof/glass/trunk area downward (see image below). This downward air flow will vary in smoothness (whether it's more laminar or more turbulent, more attached or more detached) depending on the shape of the vehicle (notice the air flow differences in the two vehicles shown below).

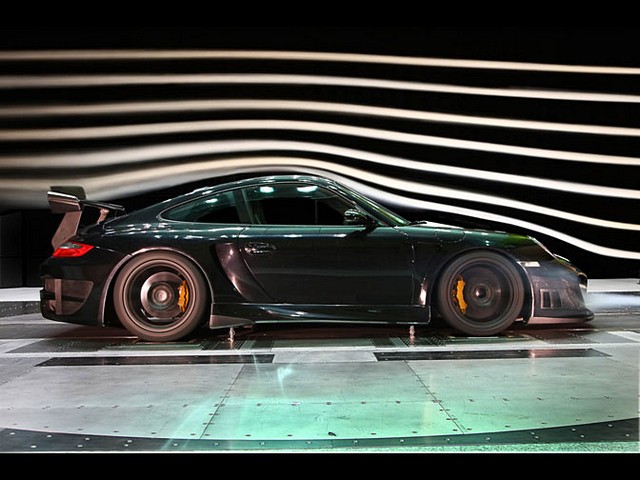
(Image from 3rd party source)
Knowing exactly what angles the air flows downward behind the roof and around the vehicle body is information that would be "nice-to-know," but in practice, we don't really need to know all the details. What we need to know is that the vehicle body has enormous effect on the air flow that reaches the airfoil. We should also know that the center section of the airfoil sees an effective AOA value greater than 0 degrees when the airfoil pitch is set to 0 degrees, and that the outer sections of the airfoil see outer-section-only AOA values around 15 +/- some value based on how the body affects the air flow. We already know from the CFD data that the outer sections of the airfoil begin to stall when the center AOA is around 10 to 12 degrees, with even greater stall at 12-13 degrees. Therefore, we can determine that the downward pitch of the airfoil should never need to be set beyond 12 to 13 degrees. In vehicles with steeper rear roof/glass/trunk slope, the airfoil pitch may never need to be set beyond 10-12 degrees. Remember, the inner or outer sections of the airfoil will begin to stall at effective AOA values of 25 to 27 degrees, with even greater stall at 27 to 28 degrees.
To re-iterate what was mentioned above, and to emphasize a few points:
1. At a center section pitch of 0 degrees, the effective AOAs of both the center and outer sections is greater than 0 degrees. --> The airfoil is already creating downforce at 0 degrees pitch.
2. At a center section upward pitch of greater than 0 degrees, the effective AOAs of both the center and outer sections will still be greater than 0 degrees up to a certain point. --> The airfoil is still creating downforce at "positive" pitch values..
3. A roof that slopes down to the trunk area gradually will allow the air to flow more smoothly (i.e. stay attached longer, be more laminar, less turbulent, etc.) than a roof that slopes down abruptly to the trunk area. --> Be very aware of how significant is the effect that the vehicle body shape has on the air flow to the airfoil when trying to determine airfoil placement and airfoil pitch.
Airfoil Setup
Setting the Airfoil Height:As a general guideline, vehicles with steeper-sloped rear roof/glass/trunk areas are better suited to use higher airfoil mounting heights (just below the roof line). Vehicles with gradually-sloped rear sections (i.e. fastbacks) can work well with lower airfoil mounting heights. There is no strict rule for this, since every vehicle application is different. The GTC-300 airfoil comes with pedestals of a recommended height for each intended vehicle application. Additional height can be achieved by using optional risers..
Setting the Angle: It is helpful to know how the vehicle handles prior to installation of the airfoil. For the initial testing with the airfoil installed, we recommend setting the airfoil pitch to 0 degrees using an angle indicator tool. Test the vehicle's front vs. rear cornering balance at speeds above 45-55mph (approx 72-89 km/h) (on a familiar track surface). If the vehicle tends to understeer (feel "tight") too much at medium-high speeds, then dial in a bit of upward pitch. If the vehicle tends to oversteer (feel "loose") at medium-high speeds, then dial in a bit of downward pitch. Try to make very small adjustments as needed between each road test, and try to focus on adjusting one thing at a time (i.e. don't change the airfoil pitch, tires pressures, and shock settings all at once)).
Initial angle setup with the airfoil pitch set to 0 degrees, as shown on the digital angle indicator:

Same as above, but with the angle indicator on top of the outer section:

Maximum Angle: We don't recommend setting the downward pitch greater than 12 to 13 degrees, even on vehicles with the most gradually-sloped rear roof/glass/trunk areas. On vehicles with steeper angles at the rear, the downward pitch should not be set greater than 10-12 degrees.
Recommended maximum angle, as shown on the digital angle indicator:

Same as above, but with the angle indicator on top of the outer section:

Conclusion
With so many vehicle configurations, track and road conditions, and weather and environment conditions, it is nearly impossible to predict exactly how an aerodynamic component will change a vehicle's performance and handling characteristics. Well-funded race teams continuously spend significant amounts of time and money to perform computer simulations, wind tunnel tests, track tests, and other research and development activities. For most folks, all these activities may not be within convenient access. Nevertheless, we can at least let the CFD data and analyses contained herein serve as starting guidelines in our quest to improve vehicle aerodynamic performance.
The information contained herein is property of APR Performance, and may not be reproduced in whole or in part without prior written consent from APR Performance.